The story behind our recent paper.
Don’t be afraid to explore crazy ideas. That is the lesson I learned from my latest publication in the Journal of Avian Biology. In this blog post, I will not only share the main results of this paper, but also the entire process behind the scenes. A nice example that the development of a paper is rarely linear.
Woodpecker Hybrids
It all started with my quest to assess the reliability of hybrid records in birds. Generally, the reliability of these records has been taken at face value. However, detailed assessments of certain hybrids in the Handbook of Avian Hybrids of the World and the Serge Dumont Bird Hybrids Database revealed that some cases are unreliable or incorrect. For example, only one out of four records of tinamou hybrids turned out to be supported by convincing evidence (see this paper for the details). That is why I decided to revisit hybrids records in several bird groups. The next group on the list were the woodpeckers. There was no particular reason for this choice. Woodpeckers are just very cool birds.
My exploration of old papers and handbooks uncovered 68 hybrid combinations of which 37 cases (54.41%) were supported by convincing evidence. In total, 88 species (36.67%) were reported to have hybridized with at least one other species. However, only 46 species (19.17%) had reliable hybrid records. These numbers indicate that many hybrid records will need to be re-evaluated. I have described an example of an unreliable case in another blog post. A putative hybrid between Chestnut-colored Woodpecker (Celeus castaneus) and Golden-olive Woodpecker (Piculus rubiginosus) could be traced back to an erroneous interpretation of a footnote in a checklist of Mexican birds. Always check the original source.
Enter Mimicry
Now what? This question popped into my head when I had finished my list of reliable woodpecker hybrids. I could publish this overview in a short paper, but I wanted to add something. Then I remembered a blog post that I wrote some time ago: “Why do Robust Woodpecker, Lineated Woodpecker and Helmeted Woodpecker look alike?” These three species belong to different genera, but look scarily similar. This impressive case of plumage convergence can be explained by the “interspecific social dominance hypothesis” which states that subordinate species will look like a dominant one to avoid attacks by the dominant species.
This phenomenon got me thinking about the relationship between hybridization and mimicry. During a quick literature search, I discovered a paper by David Pfennig and David Kikuchi in which they discuss several mechanisms that can hamper the evolution of mimicry. One interesting possibility concerns the “selective trade-off hypothesis” where opposing selective forces prevent the evolution of perfect mimicry. Looking at the relationship between hybridization and mimicry suggested a potential trade-off: selection for mimicry drives plumage convergence whereas selection against maladaptive hybridization leads to plumage divergence.
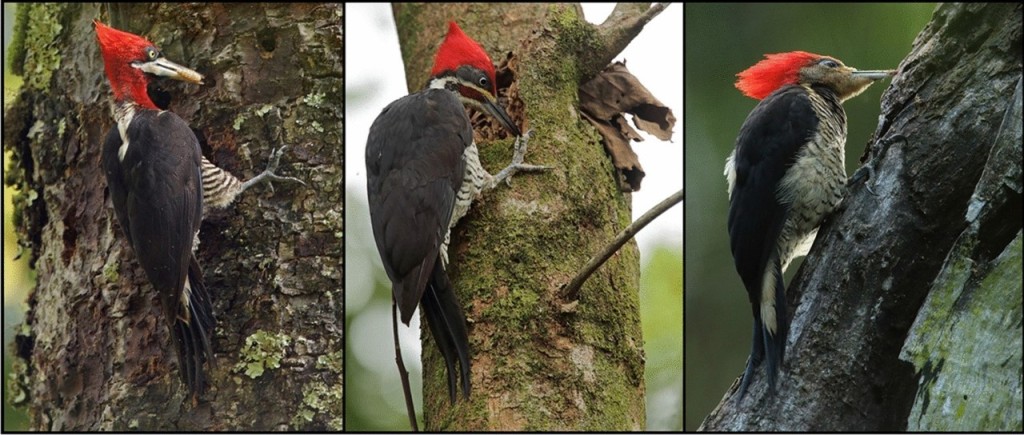
Tipping Point
To analyze the relationship between hybridization and mimicry, I collected data on mimicry complexes in woodpeckers. Next, for one of these analyses, I needed to know which woodpecker species occur in the same area. I reached out on Twitter and Michaël Nicolaï volunteered to help out. I send him my list of woodpecker species, and he provided me with an overview of sympatric species. My first Twitter-collaboration!
The analyses revealed a clear pattern. We found a clear tipping point in the probability of hybridization and mimicry at ca. 9 million years of divergence. Around this timepoint, the probability of hybridization approaches zero while the probability of belonging to a mimicry complex increases. It looked almost too good to be true. However, we highlighted that this finding is only correlational and remains to be confirmed in other taxonomic groups. We would expect different tipping points in different bird groups (depending on the timepoint when the probability of hybridization reaches zero). Nonetheless, our results suggest a selective tradeoff between evolving interspecific mimicry and avoiding maladaptive hybridization in woodpeckers.
A crazy idea that culminated in an exciting paper.

References
Ottenburghs, J., & Nicolaï, M. P. (2024). Hybridization constrains the evolution of mimicry complexes in woodpeckers. Journal of Avian Biology, e03228.
Featured image: Downy woodpecker (Dryobates pubescens) © Rhododendrites | Wikimedia Commons